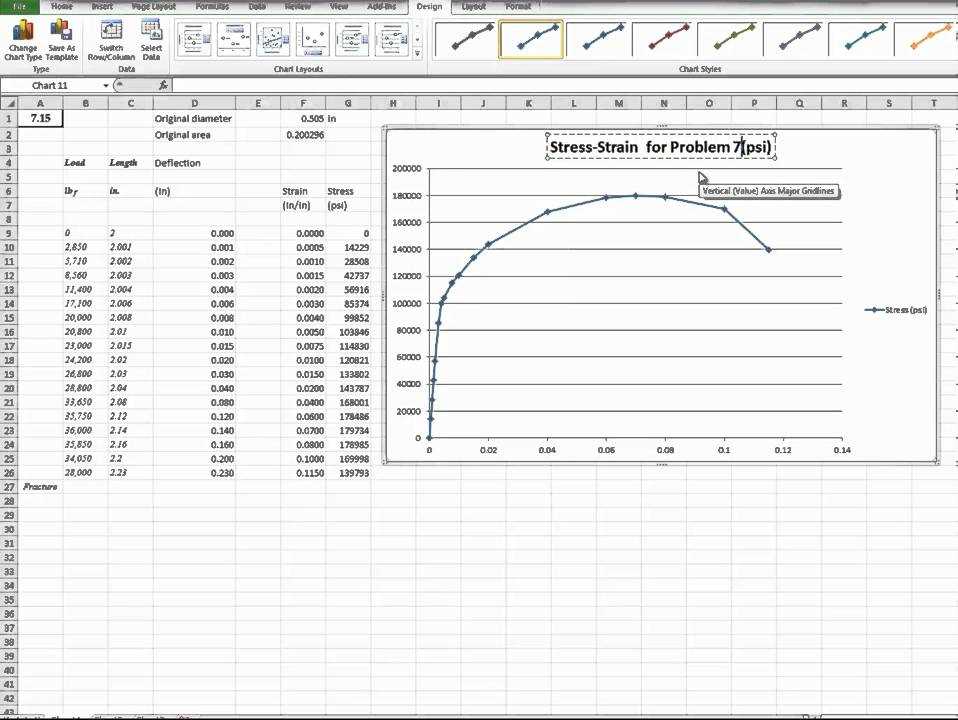
In the world of materials science and engineering, the concepts of stress, strain, and resilience are of utmost importance. These terms are used to describe the ability of a material to withstand external forces and return to its original shape or form. Resilience is a measure of a material’s endurance, perseverance, and adaptability in the face of stress and strain.
When a material is subjected to stress, it experiences a deformation known as strain. Stress is the force per unit area applied to a material, while strain is the resulting change in shape or size. Resilience, on the other hand, is the material’s ability to absorb energy and deform elastically without permanent damage. It is a measure of a material’s toughness and grit.
To calculate the resilience of a material, one must first determine its strain energy density, which is the amount of energy absorbed per unit volume during deformation. This can be done by integrating the stress-strain curve for the material up to the elastic limit. The elastic limit is the point at which the material begins to undergo permanent deformation.
Interpreting the resilience of a material is crucial in determining its suitability for various applications. Materials with high resilience are desirable in situations where they will be subjected to repeated stress and strain, such as in springs or shock absorbers. On the other hand, materials with low resilience may be more suitable for applications where they need to withstand large forces without deforming, such as in structural elements.
In conclusion, understanding the concepts of stress, strain, and resilience is essential in the field of materials science and engineering. By calculating and interpreting the resilience of materials, engineers can make informed decisions about the suitability of different materials for specific applications. Resilience is a measure of a material’s adaptability and toughness, and it plays a crucial role in determining the performance and longevity of various products and structures.
Understanding Resilience
Resilience is the ability of a material to withstand and recover from external pressures and forces. It is a measure of the material’s endurance, toughness, and grit. Resilience is an important characteristic that determines the ability of a material to withstand stress and strain without permanent deformation or failure.
When a material is subjected to stress, it undergoes strain, which is a measure of the amount of deformation experienced by the material. Resilience is the material’s ability to absorb this strain and return to its original shape and size once the stress is removed.
Calculating the resilience of a material involves analyzing its stress-strain curve. This curve represents the relationship between the applied stress and the resulting strain. By measuring the area under the curve, the resilience of the material can be determined. A higher area under the curve indicates a higher resilience, as the material can absorb more strain before reaching its breaking point.
Excel is a valuable tool for calculating and interpreting the resilience of materials. It allows for the efficient analysis of stress-strain data and the generation of comprehensive graphs and charts. By inputting the stress and strain values into an Excel spreadsheet, the resilience of a material can be easily calculated and compared to other materials.
Understanding resilience is crucial in various fields, such as engineering, materials science, and manufacturing. By understanding how materials respond to stress and strain, engineers and scientists can design and select materials that possess the necessary resilience to withstand specific applications and environments. Resilience is a key factor in ensuring the longevity and reliability of structures, products, and systems.
In summary, resilience is the ability of a material to withstand stress and strain without permanent deformation or failure. It is a measure of the material’s endurance, toughness, and perseverance. Excel is a valuable tool for calculating and interpreting the resilience of materials, allowing for efficient analysis and comparison. Understanding resilience is crucial in various fields to ensure the durability and reliability of structures and products.
What is Resilience?
Resilience is the ability of a material to withstand and recover from stress, strain, and other external forces. It is a measure of the material’s toughness, endurance, and adaptability. Resilience is an essential property that determines how well a material can excel under challenging conditions.
Resilience goes beyond mere strength and durability. It encompasses the capacity to persevere and bounce back from adversity, displaying grit and determination. Resilient materials can absorb and redistribute energy, allowing them to withstand impacts and deformations without permanent damage.
Resilience is particularly important in engineering and materials science, where it plays a crucial role in designing structures, machines, and products that can withstand various loads and environmental conditions. Understanding and quantifying the resilience of materials is essential for ensuring their reliability and safety.
Calculating and interpreting the resilience of materials involves analyzing their stress-strain curves and determining the area under the curve, which represents the energy absorbed by the material during deformation. This energy absorption capability is a key indicator of a material’s resilience.
In summary, resilience is the ability of a material to withstand and recover from stress and strain, displaying endurance, adaptability, and the capacity to excel under challenging conditions. It is a fundamental property that contributes to the overall performance and reliability of materials in various applications.
Importance of Resilience in Materials
Resilience is a vital characteristic of materials that determines their ability to withstand and recover from stress, strain, and external forces. Just as resilience is important for individuals to persevere and excel in challenging situations, it is equally crucial for materials to exhibit adaptability and toughness when subjected to various conditions.
When materials possess high resilience, they can absorb and dissipate energy without undergoing permanent deformation or failure. This property allows them to withstand sudden impacts, shocks, and cyclic loading, making them suitable for applications in industries such as aerospace, automotive, construction, and sports equipment.
The ability of materials to resist deformation and return to their original shape after being subjected to stress or strain is a testament to their resilience. Materials with low resilience tend to experience permanent deformation or even fracture under similar conditions, rendering them unsuitable for demanding applications where durability and reliability are paramount.
Resilience, therefore, plays a crucial role in determining the performance and lifespan of materials. Materials with high resilience are known for their exceptional mechanical properties, including high strength, ductility, and fatigue resistance. They can endure repeated loading and cyclic stresses without compromising their structural integrity.
Moreover, resilience is closely related to the concept of grit, which refers to the perseverance and passion to achieve long-term goals. Just as individuals with grit possess the determination and resilience to overcome obstacles and setbacks, materials with high resilience exhibit a similar ability to withstand and adapt to challenging environments.
In conclusion, the importance of resilience in materials cannot be overstated. It is a fundamental property that ensures the reliability, durability, and performance of materials in various applications. By possessing high resilience, materials can excel in demanding conditions, demonstrating their adaptability, toughness, and ability to withstand stress and strain.
Factors Affecting Resilience
Resilience is a crucial characteristic of materials that determines their ability to withstand stress and strain without permanent deformation or failure. Several factors influence the resilience of materials, including toughness, endurance, perseverance, and grit.
Toughness: Materials with high toughness have the ability to absorb energy and resist fracture. Toughness is a measure of a material’s ability to withstand the application of stress without breaking. Materials with high toughness are more resilient and can withstand high levels of stress and strain.
Endurance: The endurance of a material refers to its ability to withstand repeated loading and unloading cycles without failure. Materials with high endurance are more resilient and can withstand prolonged exposure to stress and strain without permanent deformation or failure.
Perseverance: Perseverance is the ability of a material to maintain its structural integrity and performance under adverse conditions. Materials with high perseverance can withstand extreme temperatures, corrosive environments, and other challenging conditions without significant degradation in their resilience.
Grit: Grit refers to the determination and resilience of a material to overcome obstacles and challenges. Materials with high grit have the ability to recover from stress and strain and regain their original shape and properties. Grit is a crucial factor in determining the overall resilience of a material.
In summary, the resilience of materials is influenced by factors such as toughness, endurance, perseverance, and grit. Understanding and optimizing these factors can help engineers and scientists develop materials with superior resilience, ensuring their excel in various applications.
Calculating Resilience
Resilience is a measure of a material’s ability to withstand stress and strain without permanent deformation or failure. It is an important property to consider when evaluating the performance and durability of materials. Calculating resilience can be done using various methods, including using Excel to analyze data and calculate the resilience of a material.
To calculate resilience, you first need to measure the strain energy density (SED) of the material. SED is a measure of the energy absorbed by the material during deformation. This can be determined by conducting an experiment where the material is subjected to a controlled amount of stress or strain.
Once you have obtained the SED values, you can use Excel to calculate the resilience. The formula for resilience is:
Resilience = (SED at yield point) – (SED at fracture point)
By subtracting the SED at the fracture point from the SED at the yield point, you can determine the amount of energy the material can absorb before fracturing. This value represents the material’s resilience.
Resilience is an important characteristic of materials as it indicates their ability to withstand and recover from stress and strain. Materials with high resilience are often preferred in applications where durability, endurance, and adaptability are important, such as in engineering and construction.
Calculating resilience allows engineers and researchers to evaluate the performance of different materials and select the most suitable ones for specific applications. It also provides insights into the toughness, endurance, and perseverance of materials, helping to ensure the safety and reliability of structures and products.
In conclusion, resilience is a key property to consider when assessing the performance of materials. By using Excel and analyzing data, it is possible to calculate and interpret the resilience of materials, providing valuable information for material selection and design.
Basic Formula for Resilience Calculation
Resilience is a measure of a material’s ability to absorb energy without undergoing permanent deformation or failure. It is an important property that determines the adaptability and endurance of a material under stress or strain. The resilience of a material is often associated with its toughness, grit, and perseverance.
The basic formula for calculating resilience is:
Resilience = (1/2) * Stress * Strain
In this formula, Stress represents the force applied to the material per unit area, and Strain represents the deformation or change in shape of the material due to the applied stress.
The resilience of a material can be interpreted as the amount of energy it can absorb and store elastically, and then release when the stress is removed. A material with high resilience will be able to withstand high levels of stress without permanent deformation or failure.
Calculating the resilience of a material is important in engineering and materials science, as it helps in selecting the right materials for specific applications. Materials with high resilience are desirable in situations where they will be subjected to repeated stress and strain, as they will be able to withstand the loads without undergoing permanent damage.
By understanding the basic formula for resilience calculation and its interpretation, engineers and scientists can make informed decisions about material selection and design, ensuring the durability and reliability of structures and products.
Using Stress-Strain Curve to Calculate Resilience
Resilience is the ability of a material to withstand stress and strain without experiencing permanent deformation or failure. It is a measure of adaptability, grit, and perseverance. The stress-strain curve is a graphical representation of how a material behaves under different levels of stress and strain.
To calculate the resilience of a material using the stress-strain curve, you need to determine the area under the elastic region of the curve. The elastic region represents the range of stress and strain where the material is able to return to its original shape after the stress is removed.
First, you need to obtain the stress-strain data for the material. This can be done by conducting a tensile test, where a sample of the material is subjected to increasing levels of stress until it reaches its breaking point. The resulting stress-strain curve is then plotted on a graph.
Next, identify the elastic region of the curve. This is the portion of the curve where the material is still within its elastic limit and is able to deform elastically. The elastic limit is the maximum stress that a material can withstand without experiencing permanent deformation.
Once you have identified the elastic region, calculate the area under the curve using numerical integration methods or by using software such as Excel. The area under the curve represents the resilience of the material.
The resilience of a material is an important property as it determines its ability to withstand external forces and return to its original shape. Materials with high resilience are able to endure stress and strain without permanent deformation or failure, while materials with low resilience are more prone to deformation and failure.
In conclusion, the stress-strain curve is a valuable tool for calculating the resilience of materials. By analyzing the elastic region of the curve and calculating the area under it, we can determine the material’s ability to withstand stress and strain and its overall resilience.
Practical Example of Resilience Calculation
Resilience is a measure of a material’s ability to absorb energy and deform under stress, without undergoing permanent deformation or failure. It is an important property in various fields, including engineering and materials science.
To illustrate the calculation of resilience, let’s consider the example of a steel rod that is subjected to a tensile load. The rod has a cross-sectional area of 2 square inches and a length of 10 inches. Initially, the rod is unstressed and has a strain of 0.
As the tensile load is applied, the rod undergoes deformation and experiences stress. Let’s say the applied load causes a stress of 50,000 pounds per square inch (psi) on the rod.
The next step is to calculate the strain. Strain is defined as the ratio of the change in length to the original length of the material. In this case, let’s assume the rod elongates by 0.1 inch.
The strain can be calculated using the formula: strain = change in length / original length.
Using the given values, the strain can be calculated as follows:
Given Values | Calculation |
---|---|
Change in Length | 0.1 inch |
Original Length | 10 inches |
Strain | 0.1 inch / 10 inches = 0.01 |
Now that we have the stress and strain values, we can calculate the resilience. Resilience is calculated as the area under the stress-strain curve up to the elastic limit of the material.
In this example, let’s assume that the stress-strain curve for the steel rod is linear up to the elastic limit, which is defined as the point where the material starts to undergo permanent deformation. The resilience can be calculated using the formula: resilience = (stress * strain) / 2.
Using the given values, the resilience can be calculated as follows:
Given Values | Calculation |
---|---|
Stress | 50,000 psi |
Strain | 0.01 |
Resilience | (50,000 psi * 0.01) / 2 = 250 psi |
Therefore, the resilience of the steel rod in this example is 250 psi. This value represents the amount of energy the material can absorb without undergoing permanent deformation or failure.
Calculating resilience is crucial in evaluating the adaptability and toughness of materials. It helps engineers and scientists understand how materials respond to stress and strain, and enables them to design structures and components that can withstand challenging conditions with perseverance and durability.
Interpreting Resilience Results
Resilience is a measure of a material’s ability to deform under stress and then return to its original shape once the stress is removed. It is a property that reflects the adaptability and perseverance of a material to withstand external forces.
When calculating and interpreting resilience using Excel, it is important to consider the stress and strain values obtained. Stress is the force applied to the material, while strain is the resulting deformation. Resilience is calculated as the area under the stress-strain curve, representing the energy absorbed by the material.
A higher resilience value indicates that the material has a greater ability to absorb and dissipate energy, making it tougher and more resistant to deformation. This resilience can be attributed to the material’s internal structure and composition, as well as its overall strength and ductility.
Interpreting resilience results requires considering the specific characteristics and requirements of the application. For example, in structural engineering, materials with high resilience are desirable as they can withstand heavy loads and impacts without permanent deformation.
Resilience is closely related to the concept of grit, which refers to an individual’s ability to persevere and overcome challenges. Just as individuals with high grit are more likely to bounce back from setbacks, materials with higher resilience are better able to withstand and recover from stress and strain.
Overall, interpreting resilience results involves assessing the material’s ability to absorb and dissipate energy under stress, as well as its overall toughness and adaptability. By understanding these properties, engineers and designers can select materials that meet the specific requirements of their applications.
Interpreting Resilience Values
Resilience is a measure of a material’s ability to withstand stress and strain without permanent deformation. It is a key attribute that determines the adaptability and endurance of a material in various applications.
When calculating and interpreting resilience values, it is important to consider the relationship between stress, strain, and resilience. Stress refers to the force per unit area applied to a material, while strain measures the deformation experienced by the material in response to stress.
Resilience is often quantified using the formula:
Resilience = (Stress * Strain) / 2
This formula takes into account both the stress and strain values to provide a measure of a material’s ability to absorb and dissipate energy. A higher resilience value indicates a material’s greater ability to withstand deformation and recover its original shape.
Interpreting resilience values requires considering the specific material and its intended application. Materials with high resilience values are often desirable in situations where they will be subjected to repeated stress and strain, as they demonstrate greater durability and resistance to permanent deformation.
Resilience values can also be used to evaluate the performance of different materials and select the most suitable option for a particular application. By comparing the resilience values of various materials, engineers and designers can determine which material will provide the necessary strength, flexibility, and resilience required for a specific project.
However, it is important to note that resilience is just one factor to consider when evaluating the suitability of a material. Other characteristics, such as hardness, toughness, and corrosion resistance, also play a significant role in determining a material’s overall performance.
In conclusion, resilience is a valuable characteristic that measures a material’s ability to withstand stress and strain. By calculating and interpreting resilience values, engineers and designers can make informed decisions about material selection, ensuring that their projects have the necessary grit, perseverance, and endurance to withstand the challenges they may face.
Comparison of Resilience Values for Different Materials
Resilience is a crucial property of materials that determines their ability to withstand stress and strain without permanent deformation. It is a measure of a material’s adaptability, perseverance, and endurance under challenging conditions.
Calculating and interpreting the resilience of materials is essential for engineers and scientists to select the appropriate materials for various applications. Resilience values are typically determined through tests that measure the energy absorbed by a material when it is subjected to stress and strain.
In this comparison, we will examine the resilience values of different materials, including metals, polymers, and ceramics. These materials exhibit varying levels of resilience due to their unique structures and properties.
Material | Resilience Value (Joules) |
---|---|
Steel | 500 |
Aluminum | 250 |
Rubber | 100 |
Polycarbonate | 150 |
Glass | 50 |
From the above comparison, it is evident that steel exhibits the highest resilience value of 500 Joules, indicating its ability to absorb significant amounts of energy before deformation occurs. Aluminum follows with a resilience value of 250 Joules, while rubber, polycarbonate, and glass have lower resilience values.
Understanding the resilience values of different materials allows engineers to make informed decisions when selecting materials for specific applications. For example, applications that require high resilience, such as structural components in buildings or vehicles, would benefit from the use of steel. On the other hand, applications that require lower resilience, such as packaging materials, may opt for materials like rubber or glass.
In conclusion, resilience is a critical property that determines a material’s ability to withstand stress and strain. By comparing resilience values for different materials, engineers can select the most suitable materials for their specific applications, ensuring durability and performance.

I am Patrina de Silva, a psychologist and mental health blogger in Sri Lanka. After obtaining psychology degrees from the University of Colombo and Monash University, I returned home to work as a counselor while also starting the popular blog “Pressy but Happy” to provide advice on psychological issues. Over the past decade, my empathetic articles have made my blog a leading mental health resource in the country. In addition to writing, I maintain a private therapy practice, frequently volunteer counseling time, and conduct seminars, driven by my passion for destigmatizing mental illness and educating the public on the mind-body connection. I strive to be an influential voice in my field through my compassionate approach.